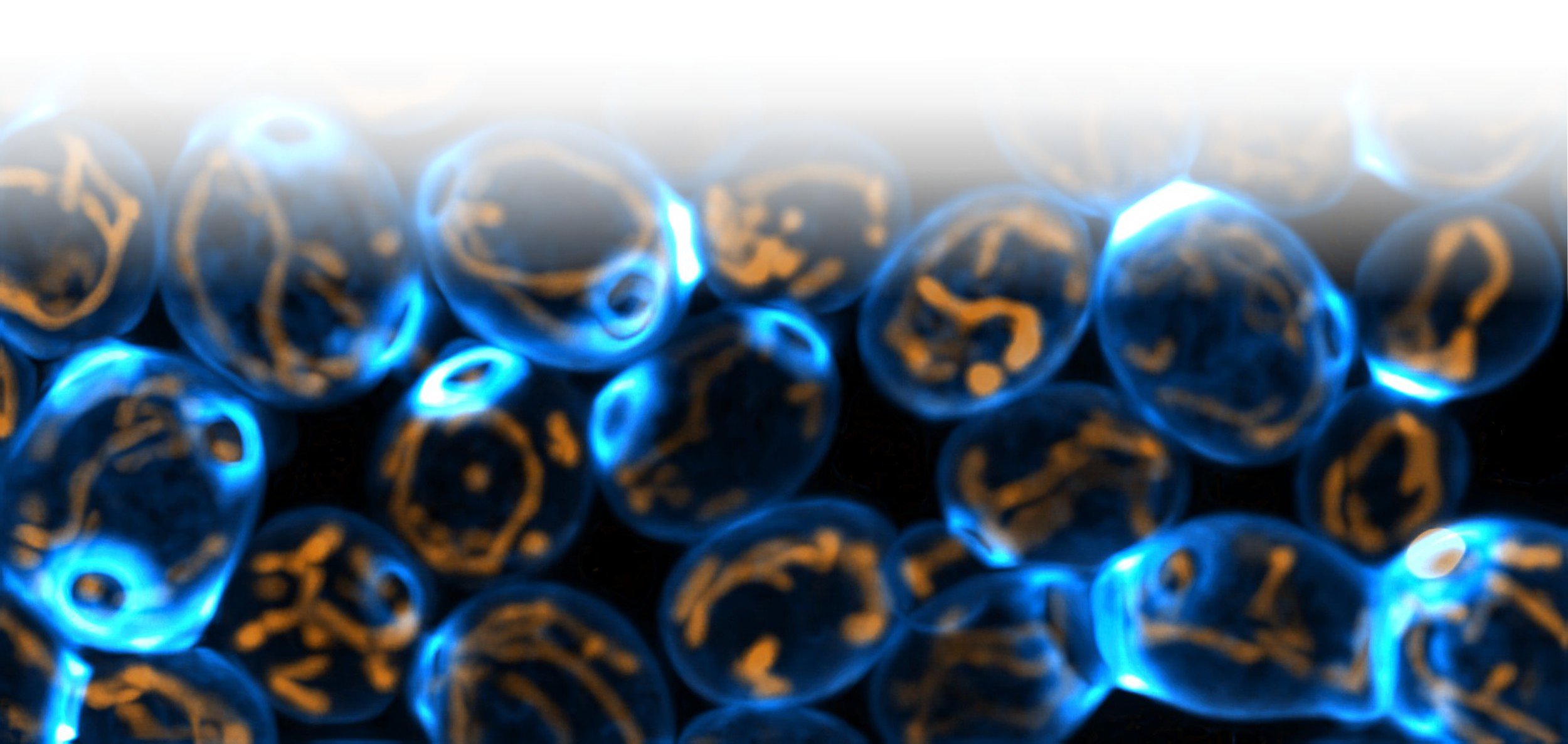
Beyond the Double Membrane: Our Research
Mitochondria, originating as separate organelles, have a complex double-membrane structure: an outer mitochondrial membrane, separated by the so-called intermembrane space from the inner mitochondrial membrane. The inside of mitochondria is termed the matrix (indeed a fancy place, but not related to the movie). This unique architecture among cellular organelles makes our research challenging, yet exciting. For instance, when investigating protein maintenance, these processes can vary depending on the mitochondrial compartment in which these proteins are located. Mitochondrial proteins can also change their compartment rapidly. This is for example the case for mitochondrially-encoded proteins that we are keenly interested in. These proteins are encoded and produced in the matrix but are transferred into the inner mitochondrial membrane during their synthesis. This means that the production of mitochondrially-encoded proteins is a dynamic process with a sophisticated spatial organisation.
– Research questions –
A major question we aim to answer in our lab is how the quality control of mitochondrially-encoded proteins is spatiotemporally organized and how this is maintained during ageing.
Given the complex organisation of mitochondrial protein production, we require a special model organism that allows us to analyse these mechanisms in detail. Our ally might surprise you:
– Our model organism –
We use the baker’s yeast (Saccharomyces cerevisiae) as our major model organism.
Mitochondria (orange) in living yeast cells (blue)
Mitochondrial physiology is evolutionarily highly conserved between yeast and higher eukaryotes like humans. Indeed, yeast has not only been an outstanding model organism for molecular studies on ageing, but a major part of our knowledge of the molecular process of mitochondrial proteostasis comes from S. cerevisiae. We apply this fantastic model and use cutting-edge molecular biological, biochemical, and cell biological methods to study mitochondrial protein quality control.
We have a state-of-the-art set of methods to modify the nuclear genome of yeast cells and use “biolistic transformations” as a special technique to modify the mitochondrial DNA. With this power of genetic engineering, we can integrate fluorescence reporters into the mitochondrial DNA to study the quality control of mitochondrially encoded proteins. With a state-of-the-art robotic system, we apply these reporters to perform genome-wide screens during ageing. Further, we aim to decipher the spatial organisation of quality control systems with biochemical approaches, and we use confocal laser scanning microscopy, flow cytometry, and immunoblotting techniques to obtain a deep mechanistic understanding of the physiological principles of protein quality control systems.
While our short-term goals are to understand the molecular principles of mitochondrial protein quality control and their changes during ageing, our long-term goal is to lay the foundation for the development of novel therapeutic strategies against age-related human diseases associated with mitochondrial protein damage, including cancer and neurodegeneration.